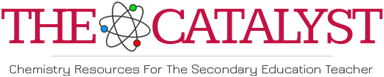
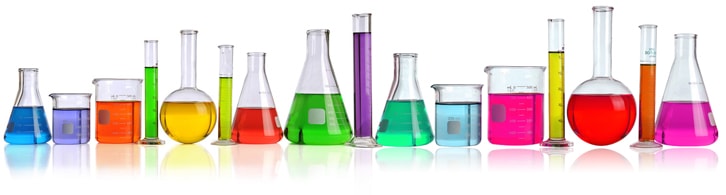
The Keto-Enol Tautomerism of 2,4-Pentanedione and Related Compounds
Bruce D. AndersonThe keto-enol tautomerism of 2,4-pentanedione is a common experiment in many undergraduate physical chemistry courses (1,2). In this experiment, the student monitors the relative amount of the tautomers using NMR spectroscopy and the equilibrium constant (keto <==> enol) can then be calculated for the reaction. The effect of different substituent groups on the equilibrium can be examined as well. The student can observe how the equilibrium position is altered as larger substituent groups are added. The proposed exercise enables the student to determine which tautomer is more stable and what happens as increasingly bulky substituent groups are added to the molecule. The student may run only one or two compounds in lab, but have time to do several others using molecular modeling software. In this way, the student will be able to more closely observe any trends in the data.
keto form | enol form | |
2,4-pentanedione |
---|
Overview of Procedure
For each compound, the keto form of the molecule is constructed and then the energy is calculated using first a molecular mechanics calculation followed by a semi-empirical one. Then the enol form of the molecule is constructed and its energy determined in the same way. The difference between the semi-empirical energies for the keto and enol tautomers is recorded and compared to those for the other molecules. While the procedure below is written specifically for Version 4.5 of HyperChem, similar procedures can be performed using other programs including: Spartan and CAChe (Project Leader).
Specific Instructions for HyperChem
To build the molecule select Build, Default Element and the Element Table will appear on the screen. Select the desired atom from the Element Table and click on the screen to position the desired atom. To make a bond, click on the atom on the screen and hold the left mouse button down until the position of the new atom is selected. When the left mouse button is released, the bond and new atom will appear on the screen. Continue to build the molecule changing atoms in the Element Table as needed. To make a double bond, point to the first atom in the bond, click and drag the cursor to the second atom. When the mouse button is released, the second bond will appear. After the molecule is constructed, select Build, Add H & Model Build from the menu. This will fill in all open valences with hydrogens and give a quick starting part for the final geometry. When constructing the enol tautomer, the Allow Ions box must be selected from the lower left hand corner of the Element Table so that a single (hydrogen) bond can be drawn between the double bonded oxygen and the enolic hydrogen.
To begin the energy calculation select Setup, and then Molecular Mechanics. When the window box appears select MM+ and click on Okay and then Okay again to recalculate the atom type. Then select Compute, Geometry Optimization, and set the gradient to 0.1 kcal/mol. The Polak - Ribiere method was used for the calculations here, but other methods should be acceptable. After the calculation is complete, select Setup, Semi-Empirical and then AM1 from the list of computational methods presented. Click on Okay and select Compute, Geometry Optimization to start the computation. Again the gradient should be set on 0.1 kcal/mol (lower gradients are achievable in a reasonable period of time if a powerful computer is used). The energy appears at the bottom left of the window when the calculation is complete. Save the file if desired and repeat for the next tautomer or molecule.
Results and Discussion
Table I shows the calculated change in energy (kcal/mol) for the tautomers of 2,4-pentanedione and several related compounds. Literature values are included where available (3). The literature values given are actually the change in enthalpy for the gas phase conversion of keto to enol for the molecules indicated. The data shows that as the size of the substituent group increases, the equilibrium shifts to favor the keto tautomer because of steric hinderance. Notice in particular the large positive values obtained when a t-butyl or phenyl group is attached to the third carbon. It is interesting to observe that when moving from an ethyl group to a propyl or n-butyl group there is no significant difference in the energy. Students should be encouraged to examine the optimized structures on the screen and determine which groups are repelling each other to produce the steric hinderance.
Table I. |
||
---|---|---|
Compound | Energy | Literature Value |
2,4-pentanedione | -3.36 | -4.66 |
3-methyl-2,4-pentanedione | -3.27 | |
3-ethyl-2,4-pentanedione | -1.25 | |
3-propyl-2,4-pentanedione | -1.32 | |
3-n-butyl-2,4-pentanedione | -1.36 | |
3-t-butyl-2,4-pentanedione | +12.44 | |
3-phenyl-2,4-pentanedione | +17.91 |
Table II shows the calculated change in energy (kcal/mol) for several esters that are structurally similar to 2,4-pentanedione. These esters also exhibit an equilibrium between the keto and enol tautomers. Students should note that attaching the bulky groups to the ester has a much less dramatic effect on the equilibrium, but the equilibrium is shifted toward the keto (reactant) as before.
Table II. |
||
---|---|---|
Compound | Energy | Literature Value |
methyl-acetoacetonate | -2.48 | -3.04 |
ethyl-acetoacetonate | -2.49 | -3.17 |
t-butyl-acetoacetonate | -1.88 | |
phenyl-acetoacetonate | -1.82 |
Further Experiments
Additional work that could be performed along these lines is to have the student take the solvent into account when calculating the energies for a single compound. The equilibrium is highly dependent on the solvent polarity, so the effects here should be dramatic. In addition, students may want to examine the effect of attaching different substituent groups (electron-withdrawing vs electron-donating) and substitution at different positions could also be studied effectively by the students.
References
- Shoemaker, D.P., Garland, C.W., Nibler, J.W., Experiments in Physical Chemistry, 6th Ed., McGraw Hill: New York, 1996, p. 446.
- Matthews, G.P., Experimental Physical Chemistry, Oxford University Press: Oxford, 1985, p. 269.
- Folkendt, M.M., Weiss-Lopez, B.E., Chauvel, J.P., True, N.S., J Phys Chem, 89, 3347 (1985).