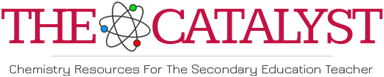
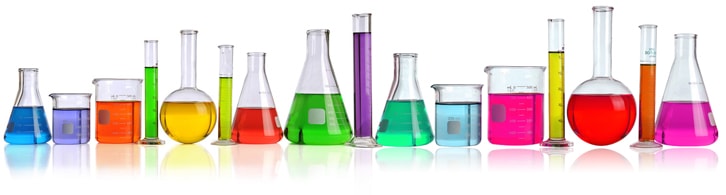
Dioxygen and Ozone: Simple Little Molecules?
Linda C. Bush
410-543-6484
Background:
Two allotropes of oxygen essential to life on our planet are dioxygen, O2, (also called "molecular oxygen") and ozone, O3. In this brief exercise we will explore the configuration and geometry of these two molecules. We will try to match what we discover through calculations with some of the characteristic properties of these molecules. For example, we know that liquified oxygen will be held between two magnetic poles. This tells us that molecular oxygen is paramagnetic. When an atom or molecule is paramagnetic it has unpaired electrons.We also know that ozone is what protects us from the most dangerous ultraviolet (UV) radiation from the sun. The "tanning rays" are UV-A radiation (315-400 nm). UV-A is thought to be less dangerous than UV-B (290-315 nm) light which causes sunburn. UV-C light is very harmful. UV-C radiation has wavelengths 100-290 nm. This radiation has a lot more energy than UV-A and UV-B, and it can cause many more harmful reactions in living tissues. We will explore the structure and predicted UV-visible spectrum of ozone.
Molecular oxygen, O2
First, draw a Lewis electron-dot Structure for an oxygen atom. There are 6 valence electrons.
Now draw the Lewis electron-dot Structure for an oxygen molecule, O2.
First draw two oxygen atoms with a bond between them. Then add in pairs of electrons until you have accounted for all 12 valence electrons
(remember there are two used to make the bond). Notice that each oxygen does not yet have an octet. Use one of the electron pairs to make a double bond, and satisfy each atom's desire for an octet.
Now that we have a starting place, let's do some molecular modeling to see what that can tell us. Draw the structure in CAChe (or other chemical modeling program) as it is shown at the right above. Remember to specify sp2 hybridization for the oxygens and "beautify" the structure. Does it look like your electron-dot drawing?
Select the MOPAC application and initiate a geometry optimization using AM1 parameters. The default setting for multiplicity should be "singlet" which constrains all electrons in the molecule to be paired. Run the calculation and record the heat of formation (Hf). You might also want to record the interatomic distance.
So, that was easy, but what's wrong with the calculation described above? Well, we already said that we know one property of oxygen is its paramagnetism. By constraining the molecule to a singlet multiplicity we haven't really calculated a paramagnetic species. To see whether the paramagnetic molecule is the favored (or ground state) electronic configuration we need to re-run the calculation with multiplicity set to "triplet". An atom or molecule which is described as a triplet has two unpaired electrons with same spin in its highest occupied orbitals. (For this system it will not matter whether you choose "triplet" or "triplet using UHF".) Run the calculation, record the Hf and interatomic distance. Likely results are shown below.
multiplicity | Heat of Formation (kcal/mol) |
bond distance (angstroms) |
---|---|---|
singlet | 0.724 | 1.087 |
triplet | -27.050 | 1.085 |
Since the triplet (with two unpaired electrons) has a lower Hf it is more stable, and thus the favored "natural" configuration for molecular oxygen. This result matches nicely with the "magnetic" behavior of liquified oxygen mentioned above.
For more advanced students, calculating the MOs of oxygen may be of interest. To do this, run the Tabulate application (if using CAChe) and request "all molecular orbitals". When the calculation is complete, the MOs may be viewed using the Visualizer application. Each surface must be opened separately. The MOs are shown below. CAChe color-codes the orbitals green/blue orbitals for filled and red/yellow for unfilled. Students can then be asked to reconcile number of nodes with the relative energy of each MO. They can also find the degeneracies and verify that filling the orbitals following Hund's Rule does indeed result in a triplet (paramagnetic) species.
Ozone, O3
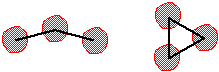
There are three oxygen atoms in ozone. Consider that there are two possible ways to connect three atoms: in a "line" and in a "ring".
Draw a Lewis electron-dot structure for each of the molecular shapes above. First draw a Lewis Dot structure for ozone where one oxygen is in the middle and the other two are attached to it. As shown in the structure centered below, adding electrons in pairs gives a molecule where the central oxygen lacks an octet. One pair of electrons must be brought over from an attached oxygen to form a double bond and satisfy all oxygen's desire for an octet. Since either of the attached oxygens can do this, there are two possible, equivalent, distributions of electrons. The two resultant resonance structures are thus obtained. Assigning formal charges to each atom reveals that these structures are zwitterions.

Use the CAChe Editor to draw a "3-in-a-row" structure for ozone. Remember to draw the double bond, specifying sp2 hybridization for those oxygens, and sp3 for the other, and also remember to assign the charges. After "beautify"-ing the structure should look like one of those shown above. If desired, run the Mechanics application to get a preliminary optimization, but remember that mechanics does not account for resonance. It would probably be just as efficient to go directly to the MOPAC application.
Select the MOPAC application and initiate a geometry optimization using AM1 parameters. The default setting for multiplicity should be "singlet" which constrains all electrons in the molecule to be paired. Run the calculation and record the heat of formation. Also record the bond lengths and the central O-O-O bond angle. You should notice that the O-O bond lengths are equal, as we would expect in a resonance hybrid.
Remember being fooled by MOPAC before? Perhaps we should not assume the singlet is preferred. Run the calculation again specifying a "triplet" multiplicity. Record values as above. Were we right the first time?
Next, draw the other possible structure for our three atom molecule. Each oxygen should be neutral and sp3 hybridized. "Beautify" and run both singlet and triplet calculations using AM1 in the MOPAC application. This time it will be important to use "triplet using UHF" to allow the electrons their greatest degree of stability possible. Again record bond distance and angles. Students may want to compare their calculated geometries with literature values. A table of likely energy data is shown below.
Molecule Shape | Multiplicity | Heat of Formation (kcal/mol) |
---|---|---|
singlet | 37.751 | |
triplet (UHF) | 56.965 | |
singlet | 111.759 | |
triplet (UHF) | 49.088 |
The above calculations have shown us the most stable structure and multiplicity for ozone. Advanced students may be interested in exploring the MOs of ground state ozone. The MOs of the ring structure may also prove interesting.
Earlier we mentioned that it is ozone which protects us from the sun's harmful UV radiation. Let's now calculate the UV-visible absorbance spectrum for our most stable structure. To obtain a predicted UV-vis spectrum in CAChe run the ZINDO application on your MOPAC-optimized ozone (use the most stable structure). When the calculation is complete, use the Visualizer application to view the predicted spectrum by choosing Electronic Spectra from the Analyze menu. Two views of the predicted spectrum are shown below. (In CAChe, the axis parameters may be changed by double clicking on the axis.)
How does the maximum absorbance correlate with the information about UV radiation given above? Note also the absorbance around 675 nm. The bluish color of condensed oxone supports this predicted absorbance.
Conclusion:
This experiment (or parts of it) can be easily adapted to the abilities of students at any level of college chemistry from General Chemistry to Physical Chemistry. There are many lessons to be learned from exploring the modeling of these two seemingly "simple little molecules". The small systems require very little time to calculate.